Physics in the Scalar Era
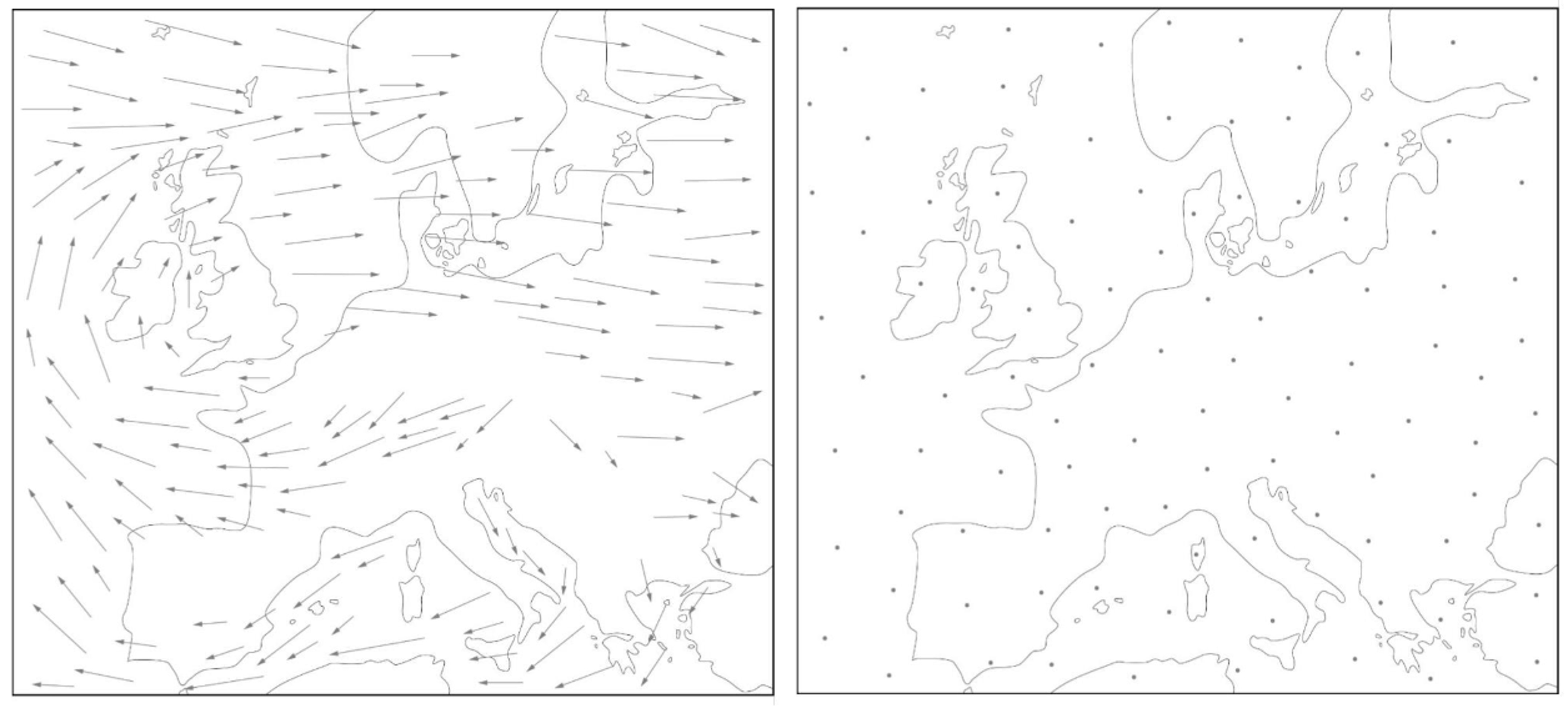
Physics for 500 years deals with forces. Forces or interactions like electromagnetism are represented by vector fields pointing from one point to another. Vectors are described by three coordinates at each point in space and time.
Scalar fields are much simpler. They represent just one number per space point. As can be seen from the figures, weather forecasts, for example, contain the scalar fields of temperature, humidity, and pressure. They are not fundamental, however, since they emerge from averaging over the microscopic properties of the air molecules. Wind maps contain vectors and are not fundamental either.
For the first time in the history of physics we go from studying fundamental vector fields of interactions to fundamental scalar fields which do not mediate interactions and do not have a source. They are omnipresent background fields which fill up the vacuum in the Universe. Such fundamental scalar fields are
● the Higgs field. It was predicted by Peter Higgs and Francois Englert in 1964 which earned them the Nobel prize in 2013. This field completes the standard model of particle physics and gives masses to the fundamental particles. This happened less than a billionth of a second after the Big Bang. The famous Higgs boson discovered at CERN in 2012 is an excitation of this field.
● the hypothetical inflation field which is believed to have caused the accelerated expansion at the very beginning of the Big Bang. We have to understand what triggered the Big Bang and how its subsequent huge inflation happened.
● the Dark Energy or cosmological constant. This is also a mysterious new field which dominates the energy content of the Universe and causes its accelerated expansion and ultimate fate .
Some physicists argue that all these scalar fields are somehow connected with each other. That is why the understanding of the nature of the Higgs field and of scalar fields in general is crucial to understand the past, present and future of the Universe. This is one of the most burning questions of fundamental science in the 21st century.
Concerning the Higgs particle the most important open questions are:
● The strengths of all fundamental interactions do not depend on the interacting particles. However, the strengths of the interactions of the Higgs field with the fundamental particles vary proportional to the masses of the particles. In order to understand how the Higgs mechanism works and generates these masses we have to test this relation as precisely and for as many different particle masses as possible!
● Check whether the Higgs particle is an elementary fundamental or a composite particle
● Check whether there are more types of Higgs bosons (charged etc.) as predicted by extensions of the standard model!
● In order to understand the Higgs field and its potential role in the early Universe, measure the interactions between the Higgs bosons!
● Check the stability of the Higgs field and hence of our Universe by measuring the masses of the Higgs boson and of the top quark as precisely as possible.
● Extensions of the standard model explain the observed low mass of the Higgs boson which is not predicted by the standard model of particle physics and propose its interactions with the mysterious dark matter dominating our Universe. Such extensions need to be investigated.
The best device to investigate all these important open questions is a new powerful electron-positron collider. Serving as a Higgs factory, it will produce a huge amount of Higgs bosons under very clean experimental conditions. A circular collider can later be extended to a proton-proton collider providing much higher energies and opening the window for discoveries beyond the standard model.